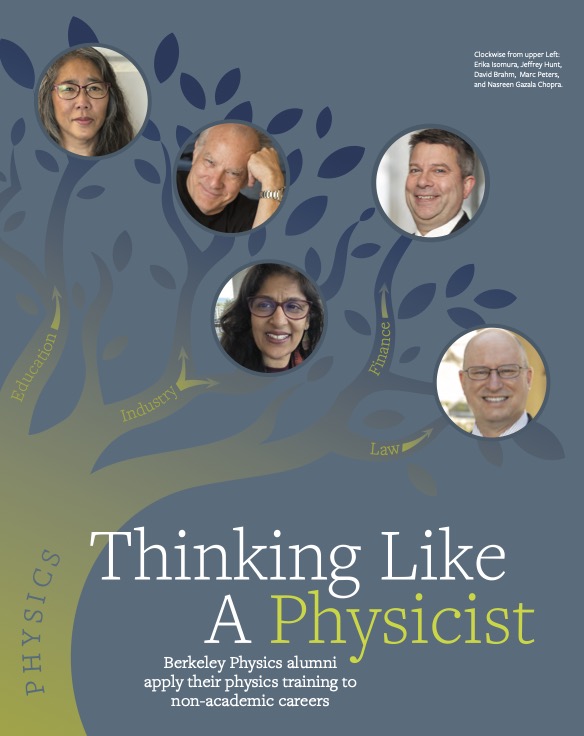
AS AN UNDERGRADUATE at UC San Diego, I knew I wanted to work in science or engineering but I wasn’t sure what I specifically wanted to do “when I grew up.” That’s why I majored in physics–it’s versatile.
After earning a PhD in physics at UC Santa Barbara, I worked for years as an academic researcher at Lawrence Berkeley National Lab (LBNL). I later veered off this career path, merging my passions for science and writing. On the surface, my physics degrees may seem unnecessary for a science journalist, given that I mostly write about medical research. But my physics training is integral to how I research, interview, and write. So, I was intrigued to speak with several Berkeley Physics alumni doing non-academic jobs to see how they apply their physics degrees.
“Physics education and training will serve you well in whatever profession you want to pursue.”
Marc Peters is an intellectual property attorney at Turner Boyd LLP. He helps clients resolve disputes related to technology, including those involving patents, trade secrets, and copyrights.
According to Peters, “thinking like a physicist” means approaching issues with the right level of abstraction, which helps determine what matters, what does not, and what is “good enough.” It also requires the curiosity to always ask “why.” These are key skills for a successful litigator.
“I was fortunate to have Professor Marjorie Shapiro as my advisor and to work with the CDF group at LBNL. They taught me how to break down any problem into solvable chunks and how to work with a group of really smart people, which I am fortunate to still do,” says Peters. “And my electronics, semiconductor, programming, and teaching experience helps me understand my clients’ technology and helps me communicate it to non-technical judges and juries.”
Peters offers some advice to current students: “Physics education and training will serve you well in whatever profession you want to pursue. You’ll learn how to learn—and that is a huge advantage in any career, inside or outside of science.”
Erika Isomura is currently a fifth-grade elementary school teacher at the Hayward Unified School District. Although she initially thought she would teach high school physics, she’s spent the last 25 years teaching all grades between second and sixth grade.
When discussing her work, she sounds like an experimental researcher. “In teaching, you don’t know in advance how things are going to turn out. So, you think about the variables, potential outcomes, and desired outcomes. And then you try things out with the kids, reflect upon what you learned, refine, and use those modifications the next day,” says Isomura. “I tease my kids that I’m a scientist experimenting on them.” She also applies this experimental approach to teaching yoga to kids.
Isomura credits her methodical planning skills to her Berkeley Physics training. Teachers need to be able to identify an end goal, as well as break that goal into manageable chunks that help students feel successful, she says.
“My work has also benefited from the classes I took at Cal. I often tell my kids about Professor Clarke, my quantum mechanics professor. His tests were open. He said it was more important that you knew how to apply the concepts than memorize or just blindly derive things. And I’ve taken that to heart,” Isomura says. “I mostly use application-level exams, rather than asking students to memorize and regurgitate something; I think that’s benefited my students.”
David Brahm recently retired as a financial portfolio manager at Geode Capital Management. As a quantitative analyst, he typically spent his days programming mathematical financial models—using databases of financial statements from several thousand companies—that aimed to maximize stock returns and minimize risk. As he describes this big data optimization problem, he is clearly still thinking like a physicist.
“To a physicist, a stock portfolio is a vector of expected returns. And every stock has some volatility, but there are also strong correlations; for example, within the airline industry. So, risk is basically a covariance matrix. And we can put these together to solve a straightforward quadratic optimization problem, where we maximize the return and minimize the risk,” explains Brahm. “Like a physicist, we make simple, useful models. However, these models can fail during an extreme event like the pandemic.”
Jeffrey Hunt is a Senior Technical Fellow at the Boeing Company. He is essentially a professor in an international company. He publishes, gets patents, gives talks, and interfaces between the technical and managerial sides of the company—always pushing the forefronts of their technology.
According to Hunt, Berkeley Physics training contributed to his success in industry. “I learned how to see the big picture, be creative, and solve problems,” he says. “My training was also very broad—optics, electronics, computer algorithms, and more—which has enabled me to reinvent myself, move around, and advance at Boeing. A physics degree is the liberal arts degree for the new millennium.”
Hunt also appreciates that his Berkeley Physics group included students and postdocs from around the world, preparing him for global collaborations.
Nasreen Gazala Chopra is the Vice President of Systems Engineering and Sustainability at Applied Materials. She spends most of her time in meetings, where she relies on the fundamentals that she learned at Berkeley Physics to successfully understand and engage in technical discussions.
“Physics also helped me think more clearly and become a stronger problem solver,” she says. “Its generality helped me get an entry ticket to jobs. And I get a lot of respect as a capable person because physics is challenging.”
Since getting her PhD in physics at Berkeley, Chopra has worked in various roles, industries, and companies, including a Silicon Valley startup, Apple, the solar industry, and the semiconductor industry. She is now excited to share this industry experience as a new member of Berkeley’s College of Letters and Science Advisory Board.
“I’m looking forward to brainstorming with my fellow board members on how we can enrich the students’ curricula and experiences,” she says. “Berkeley has a reputation for being a leader in change. I’m interested in having a frontline seat to see how that’s being implemented.”
This is a reposting of my magazine feature courtesy of UC Berkeley’s 2023 Berkeley Physics Magazine.